Computational evaluation of impact insulation of wood intermediate floors
Intermediate floors are a key building component when it comes to the competitiveness of wood apartment buildings. The sound insulation of an intermediate floor is determined mainly by impact sound insulation, rather than the airborne sound insulation. The development of wood intermediate floors for apartment buildings has long been a case of trial and error based on experimentation and measurement. This is neither cost-effective nor particularly fast. The developed parametric calculation model not only provides accurate results, it can also be used to explore different structural options quickly and to optimise the structure.
Text and figures: Pekka Latvanne, Mikko Kylliäinen, A-Insinöörit
The article has been published in Wood magazine 3/2019.
Read the article in Finnish: Puuvälipohjien askelääneneristävyyden laskennallinen arviointi
Intermediate floors are subject to stiff requirements, which makes them a key building component when it comes to the competitiveness of wood apartment buildings. Requirements are set for load-bearing capacity, deflection, vibration, fire resistance, tightness, and airborne and impact sound insulation [1], among others. In practice, impact sound insulation is more important than airborne sound insulation as a the determining factor: when the intermediate floor meets the requirements for the weighted standardised impact sound pressure level L’nT,w, the requirements for the standardised sound pressure level difference DnT,w that measures airborne sound insulation are usually also met [2, 3].
Impact sounds are a variety of residential sounds produced by impacts on the floor. Examples include objects falling on the floor, children playing, the moving of furniture and the sounds of footsteps. An apartment’s sound insulation, of which impact sound insulation is a part, has a significant effect on living comfort and health. [4]
Wood is light compared to concrete (density about 500 kg/m3). As a result, a panel-like wood layer’s airborne sound insulation is about 15 dB weaker than that of a corresponding concrete layer. What this means for wood apartment buildings is that the sound insulation between apartments cannot be based on the mass of the wood and that the transmission of sound from one space to another through space-separating structures cannot be reduced by making the joints of these structures rigid. Furthermore, the ratio of density and stiffness for wood is such that the sound insulation of a panel-like wood product is weaker than that of a corresponding gypsum board, which has a density-to-stiffness ratio that has been optimised for sound insulation. As a result, the wood structures used in construction are layered one way or another with an air gap completely or partially filled with sound-absorbing material. In addition, panels may consist of several layers. For example, one or more layers of gypsum board are typically mounted on the surface of CLT panels. [5]
Due to the complexity of the structures, there are currently no calculation models available for calculating the impact sound insulation of wooden intermediate floors, and the assessment of impact sound insulation is largely based on information derived from experience. Calculation models have been developed for calculating the impact sound insulation of massive intermediate floors [6], but they are poorly suited for evaluating the impact sound insulation of wood intermediate floors [7]. This study aimed to develop a simple parametric computation model that can be used to quickly and cost-effectively determine the L’nT, w and the impact sound levels of a wood intermediate floor by frequency band.
The study was divided into two parts: the first part investigated which structural features of a wood intermediate floor affect its impact sound insulation. This section focused in particular on identifying the factors that have a significant impact and those that are of minor importance. The study is based on an extensive review of literature. The impact sound levels of 148 wood intermediate floors measured in a laboratory served as the source material [7, 8].
Table 1. The most significant factors affecting the impact sound insulation of wood intermediate floors, as well as factors that were found to have no effect or a detrimental effect on impact sound insulation.

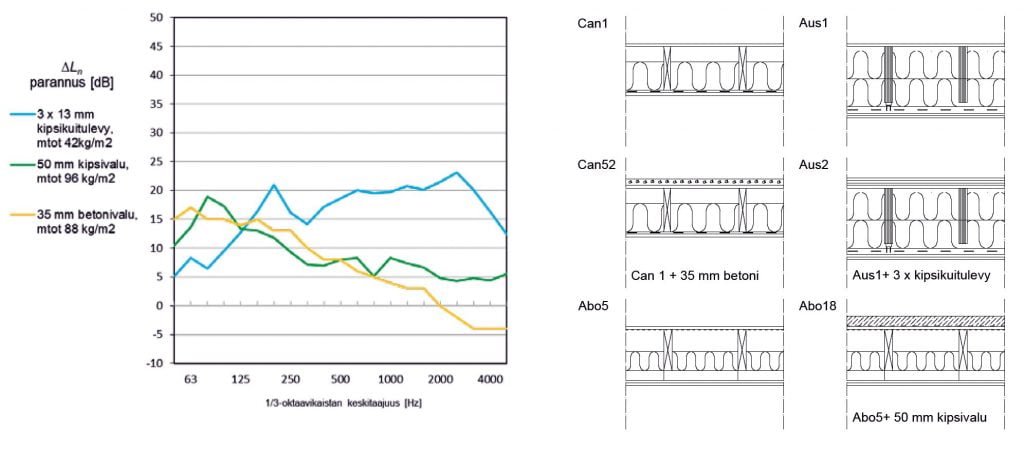
In the second part of the study, a parametric calculation model was developed. The model was based on the factors identified in the literature review as significantly influencing the impact sound insulation and on theoretical calculation models on impact sound insulation. The developed calculation model was validated by comparing the obtained calculation results to the measurement results [9, 10]. With the issuing of the Decree on the Acoustic Environment of Buildings [3], the range of impact sound insulation measurements has been extended from the traditional 100 Hz average frequency to a lower 50 Hz average frequency, as recent information suggests that the impact sounds occurring in this lower frequency range can have disruptive effects in some situations. The developed calculation model also works in this lower frequency range.
Factors affecting the impact sound insulation of a wood intermediate floor
The literature review [8] identified a number of factors affecting an intermediate floor’s impact sound insulation. Table 1 shows the most significant and non-significant or detrimental factors for the impact sound insulation of a wood intermediate floor.
Increasing the mass of an intermediate floor deck and suspended ceiling improves the impact sound insulation of the intermediate floor. The lightness of structures is an advantage of wood construction over traditional massive structure solutions [11-14], so mass increments should be optimised not only in terms of impact sound insulation but also in terms of production and cost efficiency. The mass of an intermediate floor can be increased with various slab and panel structures, either in floating form or directly mounted onto the deck plate. The properties of the floating structure’s mounting platform significantly influence how much the floating floor is able to improve the impact sound insulation [15, 16]. The challenge for cast surface structures is their required moisture management and drying time, which slows down production both on site and in factory conditions.Figure 1 illustrates the effect of panel and cast layers directly mounted on the intermediate floor deck on the impact sound insulation. The improvement caused by the mass increase is remarkable, especially at low frequencies.
The impact force excitation against the intermediate floor surface propagates through the structure as both framework sound and airborne sound [17-19]. As the structure borne sound propagating through structures is a particularly significant sound path, the impact sound insulation of a wood intermediate floor is largely based on the energy loss at the joints of the structural layers [12, 20, 21]. According to the literature review, the suspended ceiling’s joint with the load-bearing beam and the surface slab’s joint with the deck plate are the most significant joints in wood intermediate floors [8]. Figure 2 illustrates the improvements caused by different types of suspended ceilings on the beam intermediate floor. The flexible attachment of a suspended ceiling with resilient channels significantly improves its impact sound insulation compared to a suspended ceiling directly attached to the load-bearing beams. This is because the framework structure borne sound passing through the beams is effectively suppressed in the flexible joint.
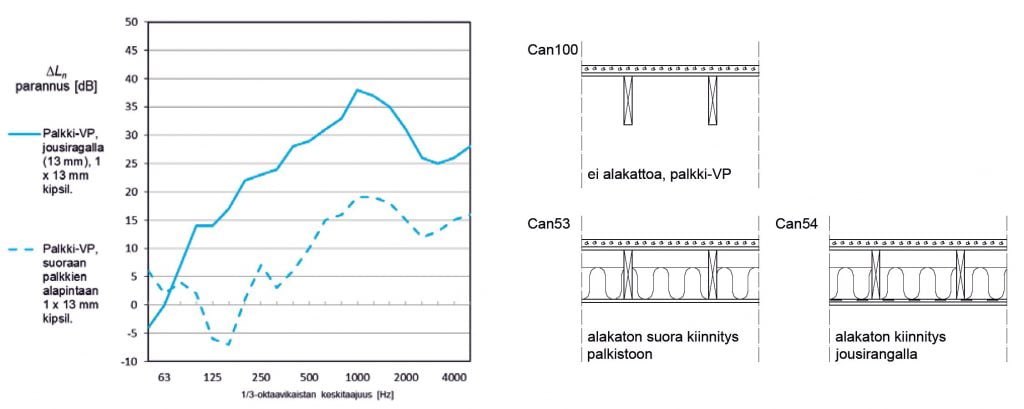
The floor covering dampens the impact on the floor surface and thereby also the structure borne sound transmitted to the entire structure [22]. Improvements caused by the impact sound insulation of floor coverings are strongly dependent on the inspected frequency and on the type of intermediate floor on which the floor covering is mounted [23]. Improvements measured for floor coverings on heavy concrete slabs cannot be used directly to estimate the improvement for wood intermediate floors. There are also differences between intermediate floors with light structures. The same floor covering produces different improvements [8, 15] on a thick CLT panel than on a thin floor gypsum board. Improvements caused by commonly used floor coverings on wood intermediate floors starts at about 250 Hz [17, 24, 25]. Tampere University of Technology has studied the effect of floor coverings on impact sound insulation on both wood and concrete intermediate floors. [4, 23] Figure 3 illustrates the improvement caused by a vinyl floor covering on impact sound insulation when mounted on different wood intermediate floors. The substructure influences the improvement at frequencies above 500 Hz in particular.
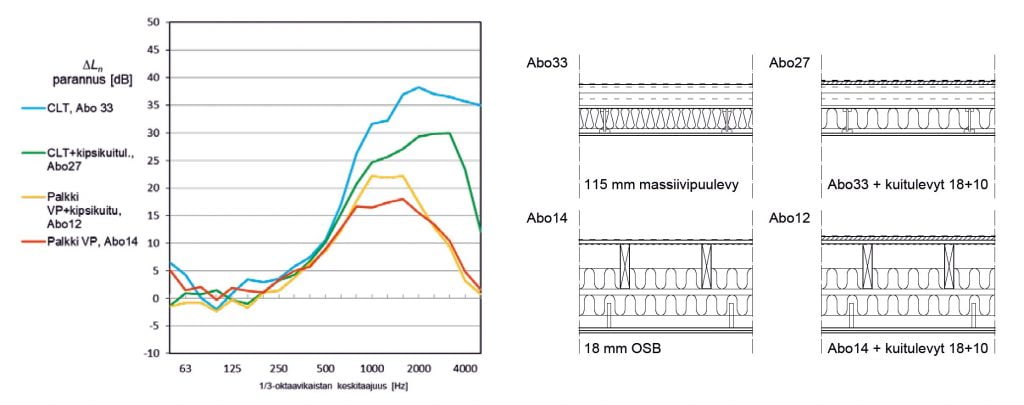
Even a thin absorption material layer inside beam-based intermediate floors significantly improves impact sound insulation [8]. The absorption material has a strong dampening effect on the airborne sound propagating inside the intermediate floor and on the standing waves in the free air space. The overfilling of air space inside an intermediate floor has not been found to have a significant effect on the improvement of impact sound insulation [8, 17]. With CLT structures, the absorption material in the airspace above a suspended ceiling was also found to dampen the sound field of the airspace. Figure 4 illustrates a comparison of the effect of different absorption material thicknesses on the impact sound insulation of an intermediate floor. The largest relative improvement is achieved by applying a thin layer of absorption material to a completely empty air space.
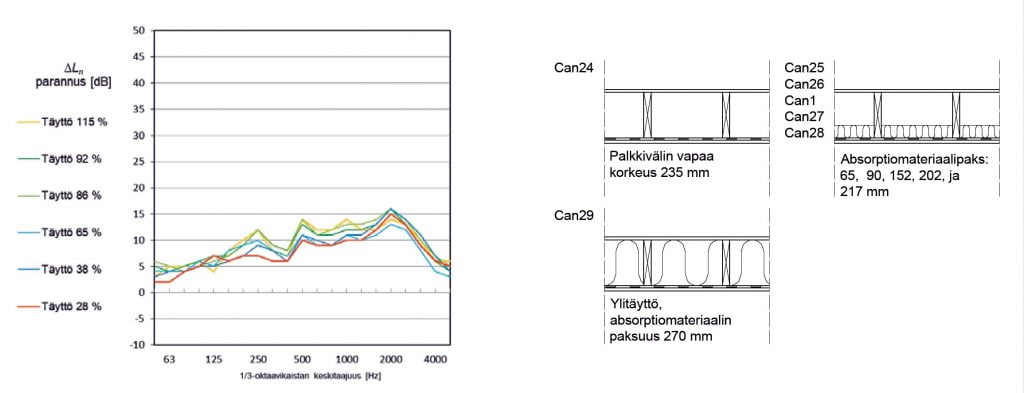
Calculation model for impact sound insulation
There have been no validated calculation methods or software available for the design of impact sound insulation of wood intermediate floors. [8] The department of Acoustic design of A-Insinöörit has developed a method for calculating impact sound insulation that is suitable for design work and product development. The method is based on an extensive literature review of parametric impact sound insulation measurements of wood intermediate floors [8] and on research and development by the A-Insinöörit acoustical design unit. The calculation model and the results of its validation have been presented previously at the international Baltic-Nordic Acoustic Meeting 2018 conference in Iceland in spring 2018 and will also be presented at the upcoming 2019 seminar on Building Physics in Tampere [9,10]. Validation was performed by comparing the impact sound levels Ln and impact sound level values Ln,w and L´n,w+CI,50-2500 determined by the calculation method to the measurement results of corresponding structures. The comparison included 28 intermediate floor structures measured in the laboratory and in residential buildings. The calculation model’s calculation steps are shown in the flow chart in Figure 5. In steps 1-3, the results are calculated in 1/3-octave bands in the 50-5000 Hz frequency range.
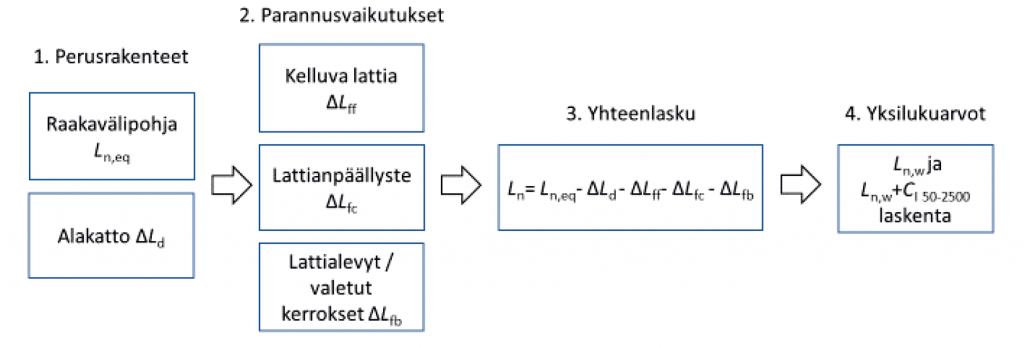
In the first step, the impact sound pressure level Ln for an unfinished intermediate floor and the improvement effect ΔLd of the suspended ceiling are calculated. In this case, the term unfinished intermediate floor refers to either a load-bearing beam and a deck attached thereto or to a load-bearing massive wood panel, such as CLT. The second step evaluates the effect of the possible floating floor ΔLff, the panel layers above the unfinished intermediate floor ΔLfb and the floor covering ΔLfc on impact sound levels. Improvements can be estimated either computationally or with measurement data [10]. Improvements caused by the panel layers mounted on the deck are combined with the computed impact sound level value of the unfinished intermediate floor, as per W. Scholl et al. [26, 27]. From the impact sound pressure levels Ln, calculated in 1/3-octave bands, the single number quantities Ln,w and Ln,w + CI,50-2500 can be determined [28]. Figure 6 illustrates the measured and calculated impact sound levels of two intermediate floors in 1/3-octave bands.
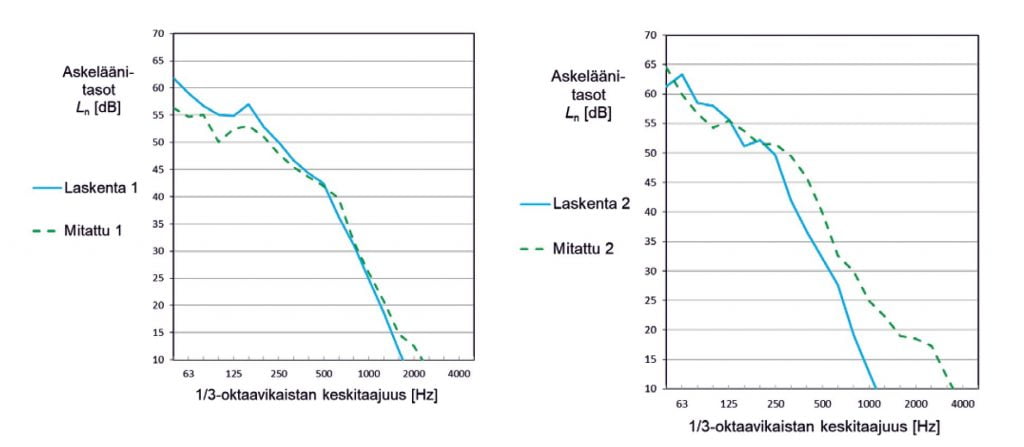
Based on the validation, the calculation results for the unfinished intermediate floors corresponded well to the measurement results across the entire frequency range studied. In the validation, it was found that the results of the calculation for CLT structures were highly dependent on the initial values of the material parameters used in the calculation. During the validation of entire intermediate floor structures, it was found that the results of the calculation model correspond best to the measurement results in the frequency range of 50-1000 Hz. The uncertainty of the calculation model increases as the structure becomes more complex. However, energy losses at the interfaces and inside of materials remain a significant factor creating uncertainty. Further development of the calculation method will require more information on the behaviour of floor coverings on wood intermediate floors as well as improved force and power calculations for unfinished intermediate floors. [10]
Using the calculation model
In Finland, the intermediate floors of wood apartment buildings have long been constructed with a floating floor attached one way or another to the load-bearing wood structure and a flexibly suspended ceiling [2]. This policy is backed by a very recent international research report, which states that without a floating floor, a wood intermediate floor structure will never have acceptable impact sound insulation [29].
The research literature describes experiments by researchers in the late 1990s where they determined the effect of floating floors and suspended ceilings on impact sound levels [30-32]. In practice, the effect of suspended ceilings on impact sound insulation was found to be greater than that of floating floors [31]. However, the experiments by the researchers did not lead to the development of an intermediate floor where the floating floor or suspended ceiling would have been left out. On the other hand, the natural frequency of a floating floor also results in high impact sound levels at low frequencies, generally below 100 Hz [33].
Source [34] cites a project that developed a wood intermediate floor that meets the current requirements for impact sound insulation [3] without the need for a floating floor structure. The acoustic performance of the intermediate floor is based on the mass of the deck plate and the flexible suspension of the suspended ceiling. The structural layers of the intermediate floor were defined by a parametric computational model developed by A-Insinöörit. The model was also able to very accurately predict the impact sound level of the intermediate floor.
The development project as a whole shows that the development of wood structures can benefit from the latest results of research and development projects. In addition, the results of the project show that it is possible to further develop wood intermediate floors for better acoustical performance and cost effectiveness. In this development work, the parametric impact sound insulation calculation model is a useful and effective tool.
Sources
1. Keronen, A. (toim.). 1998. Puukerrostalot: rakenteet, Tampereen teknillinen korkeakoulu, talonrakennustekniikan laboratorio, julkaisu 85, Tampere.
2. Kylliäinen, M. 1998. Ääneneristys. Teoksessa: Keronen A. (toim.), Puukerrostalot: rakenteet, Tampereen teknillinen korkeakoulu, talonrakennustekniikan laboratorio, julkaisu 85, Tampere, s. 59–74.
3. Ympäristöministeriön asetus rakennuksen ääniympäristöstä 796 / 2017
4. Kylliäinen, M. 2019. Rating the impact sound insulation of concrete floors with single-number quantities based on a psychoacoustic experiment. Tampere, Tampere University Dissertations 93.
5. Kylliäinen, M., Latvanne, P., Kuusinen, A. & Kekki, T. 2017. Puukerrostalojen ääneneristys –asiantuntijaselvitys. Joensuu, Karelia-ammattikorkeakoulu, Karelia-ammattikorkeakoulun julkaisu C44. 77 s.
6. EN ISO 12354-2:2000. Acoustics – Estimation of acoustic performance of buildings from the performance of elements – Part 2: Impact sound insulation between rooms. Brussels, European Committee for Standardization, 33 p.
7. Latvanne, P, Kylliäinen, M. 2015. Puuvälipohjien akustiset ominaisuudet. Rakennusfysiikka 2015 –seminaari, Tampere, 20.-22.10., Tampereen teknillinen yliopisto ja Suomen Rakennusinsinöörien Liitto RIL ry, s. 567-571
8. Latvanne, P. 2015. Puuvälipohjien akustiset ominaisuudet ja laskentamallit. Diplomityö, Tampere, Tampereen teknillinen yliopisto, Rakennustekniikan laitos, 165 s.
9. Latvanne, P., Kylliäinen, M., Kovalainen, V., Lietzén, J. 2018. An engineering method for the calculation of impact sound insulation of wooden floor constructions, BNAM 2018, Iceland Reykjavik 15-18 April 2018
10. Latvanne, P., Kylliäinen, M., Kovalainen, V., Lietzén, J. 2019. Puuvälipohjien akustiset ominaisuudet Parametrinen laskentamalli puuvälipohjien askelääneneristävyyden arviointiin. Rakennusfysiikka 2019–seminaari, Tampere, 20.-22.10., Tampereen teknillinen yliopisto ja Suomen Rakennusinsinöörien Liitto RIL ry
11. Brunskog, J. 2002. Acoustic excitation and transmission of lightweight structures. Doctoral Thesis. Sweden, LTH, Lund University, Engineering Acoustics, Report TVBA-1009.
12. Craik, R. J. M. 1998. Structure-borne sound transmission in lightweight buildings. Proceedings of ICA 1998, Seatle, USA. pp. 1387-1388.
13. Craik, R. J. M. and Galbrun, L. 2005. Vibration transmission through a frame typical of timber-framed buildings. Journal of Sound and Vibration, vol. 281(3-5), pp. 763-782.
14. Walk, M. and Keller, B. 2001. High Sound insulation wooden floor system with granular filling. Proceedings of International Congress of Acoustics 2001, Rome, Italy.
15. Warnock, A. C. C. 2000. Impact Sound Measurements on Floors Covered with Small Patches of Resilient Materials or Floating Assemblies. Canada, Institute for Research in Construction, Report IRC-IR-802
16. Zeitler, B., Sabourin, I., Schoenwald, S. and Wenzke, E. 2012. On reducing low frequency impact sound transmission in wood framed construction. Proceedings of Inter Noise, 19-22nd Aug. 2012, New York City, USA, pp. 6653-6662.
17. Warnock, A. C. C. and Birta, J.A. 2000. Detailed report for consortium on fire resistance and sound insulation of floors: Sound transmission and impact sound insulation data in 1/3 octave bands. Canada, National Research Council Canada, Institute for Research in Construction, Internal Report IR-811., 319 p.
18. Bradley, J. S. and Birta, J. A. 2001. A Simple model of the sound insulation of gypsum board on resilient supports. Noise Control Engineering Journal, vol. 49(5), pp. 216-223
19. Zeitler, B., Nightingale, T. R. T. and Schoenwald, S. 2009. Effect of floor treatments on direct impact sound pressure level. Canada, National Research Council Canada, Institute for Research in Construction, Report NRCC-53563
20. Bodlund, K. 1985. Alternative reference curves for evaluation of the impact sound insulation between dwellings. Journal of Sound and Vibration, vol. 102(3), pp. 381-402
21. Mayr, A. R. and Nightingale, T. R. T. 2007. On the mobility of joist floors and periodic rib-stiffened plates. Canada, National Research Council Canada, Institute for Research in Construction, Report NRCC-49694
22. Chung, H., Dodd, G., Emms, G., McGunnigle, K. and Schmid, G. 2006. Maximizing impact sound resistance of timber framed floor/ceiling systems, Volume 1. Australia, Forest and wood products research and development corporation, Project No. PN04.2005.
23. Lietzen, J., Miettinen, J., Kylliäinen, M. 2018. Measurements of Impact Force Excitation on Wooden Floors, Euronoise 2018, 27-31. May 2018, Heraklion, Creece
24. Forssén, J., Kropp, W., Brunskog, J., Ljunggren, S., Bard, D., Sandberg, G., Ljunggren, F., Ågren, A., Hallström, O., Dybro, H., Larsson, K., Tillberg, K., Jarnerö, K., Sjökvist, L. G., Östman, B., Hagberg, K., Bolmsvik, Å. Olsson, A., Ekstrand, C. G. and Johansson, M. 2008. Acoustics in wooden buildings, State of the art 2008. Sweden, Technical Research Institute of Sweden, Report 2008:16.
25. Hopkins, C. 2007. Sound Insulation. 1st Edition, Elsevier Ltd
26. Scholl, W., Lang, V., V. Wittstock. 2011. Rating of sound insulation at present and in future. The revision of ISO 717. Acustica united with Acta Acustica, 97, 686–698.
27. Scholl, W., Revision of ISO 717. 2011. Why not use impact sound reduction indices instead of impact sound pressure levels?, Acustica united with Acta Acustica 97, 503–508.
28. EN ISO 717-2:2013. Acoustics – Rating of sound insulation in buildings and of building elements – Part 2: Impact sound insulation. Brussels, European Committee for Standardization, 2013
29. Villot, M. et al. (eds.). 2012. Net-Acoustics for timber based lightweight buildings and elements, COST Action FP0702 E-Book, Brussels.
30. Keronen, A., Kylliäinen, M. 1996. A beam-to-column frame system from LVL, Proceedings of the first workshop “Timber frame housing in Europe – Current status and development”, Stuttgart, October 3-4, pp. 51–62.
31. Keronen, A. and Kylliäinen, M. 1997. Sound insulating structures of beam-to-column framed wooden apartment buildings, Tampere University of Technology, Laboratory of Structural Engineering, Publication 77, Tampere.
32. Keronen, A. and Kylliäinen, M. 1998. Structural solutions to improve sound insulation in timber frame houses, COST Action E5 workshop “Acoustic performance of medium-rise timber buildings”, Dublin, December 3–4.
33. Kylliäinen, M. 2008. Askelääneneristyksen mittausmenetelmän ongelmien tausta, Rakenteiden Mekaniikka 41(2008), s. 58–65.
34. Kylliäinen, M., Björkman, J. & Hakkarainen, J. 2015. Akustisesti toimivan kustannustehokkaan puuvälipohjan kehittäminen. Rakennusfysiikka
2015. Tampere, 20.–22.10., Tampereen teknillinen yliopisto ja Suomen Rakennusinsinöörien Liitto RIL ry, s. 573–578.
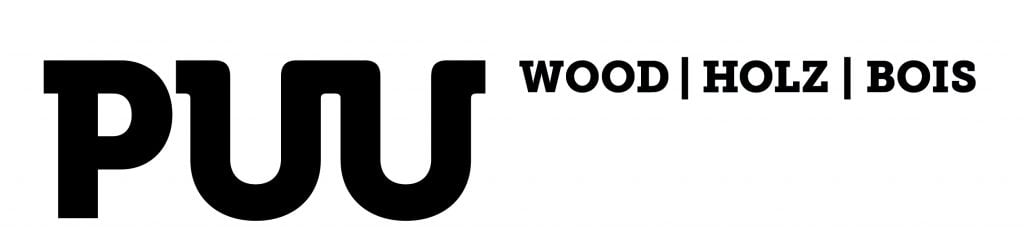