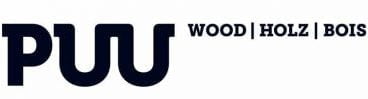
Modern computing technologies offer new acoustic design opportunities for wooden schools
Public wood construction is gaining ground. In terms of raw numbers, wooden schools have been the most common public building constructed in recent years. Design techniques for sound insulation in wood structures have also advanced rapidly. Designers can now verify the acoustic performance of their solutions at their desktop without time-consuming laboratory measurements or experimental construction.
Text: Mikko Kylliäinen, Pekka Latvanne and Antti Mikkilä
Photographs: A-Insinöörit, Hannu Rytky, Aki Rask
Read the article in Finnish: Nykyaikaiset laskentateknologiat luovat uusia mahdollisuuksia puukoulujen akustiikkasuunnitteluun
Wood has established a foothold for itself in recent years as a building material in public buildings, especially educational facilities. This is thanks to Finnish wood construction promotion programs in particular and municipal targets for carbon footprints, etc. Municipalities have taken concrete steps in their building zoning decisions, with many reserving significant areas for wood construction.
In wooden educational facilities, sound insulation and the resulting sound environment for classrooms must be designed and built for teaching and learning. This is required by the Finnish Ministry of the Environment’s decree 796/2017 on building sound environments, regardless of building material [1]. To enable effective learning, the sound conditions in a classroom need to allow listeners to distinguish between different speakers. Noises from other rooms must be prevented from interfering with the concentration of students and teachers. In “open office” type classrooms, these good sound conditions are created with room acoustic attenuation and a masking sound system [2].
Just like acoustic design, the architectural design of educational facilities is based on functional goals. Facilities must provide a healthy, safe, and comfortable learning environment regardless of building material. A typical characteristic of wooden educational facilities is the use of wood as an interior element, and wood surfaces are often left in plain sight as much as possible [3]. For example, the log structures in the Lempäälä Kanava school lobby area are visible as an interior design element (Figure 1).
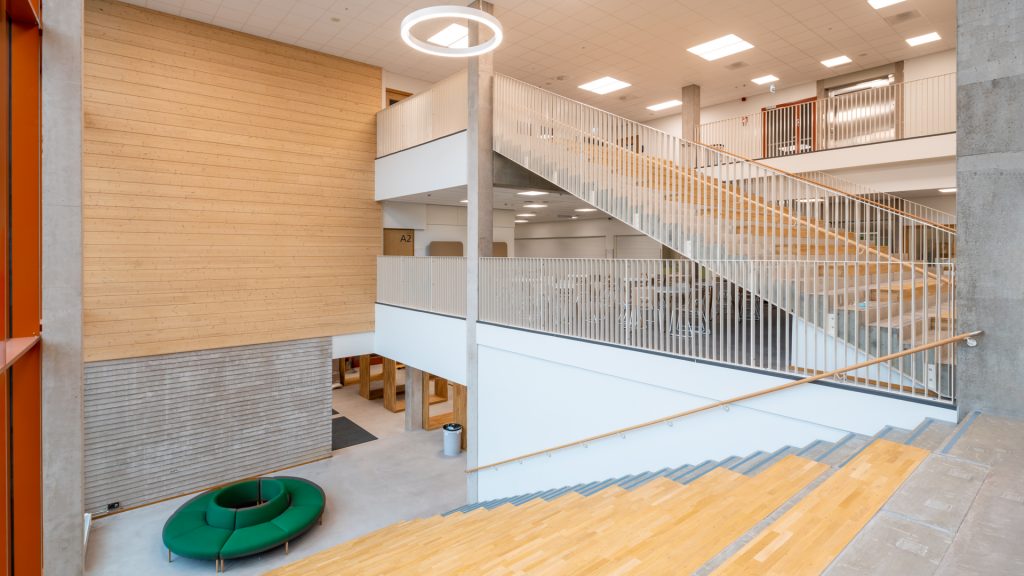
Finnish industrial wood construction is still very much under development, which is why building design processes often involve developing and brainstorming new structural solutions. This development work requires acoustic designers to evaluate the impact of different design solutions on issues such as sound insulation. The work is usually demanding because established concrete structure calculation methods and measurement results do not apply to the sound insulation calculations for wood structures. Unlike with concrete, the dimensions of wood structures are also typically determined by other factors than load-bearing requirements.
As wood construction continues to increase in public projects, the calculation methods for the acoustic properties of wood structures improve and more data is available on acoustic behaviour [for example, 4-7]. This article presents a variety of factors that influence the designs of wooden educational facilities and also takes a look at the new computing technologies for designing the sound insulation of these buildings.
Frame systems for wooden educational facilities
Acoustic designers and their expertise play an important role in the early stages of wood construction projects when the frame system and structure types are selected. The typical frame systems for wooden buildings include rigid, solid wood, log and pillar-beam [8–9]. Wood construction aims to reach a high degree of prefabrication, which in turn speeds up construction on site. In practice, a high degree of prefabrication means large CLT panels and element based rigid frames. This ensures the structures remain uniform across several spaces. In CLT and rigid frame buildings, the partitioning walls can act as load-bearing and stiffening structures.
Figure 2 shows the Hopealaakso daycare centre, which has a CLT frame. Compared to concrete structures, CLT elements are light, which means they can be lifted into place with a light mobile crane.
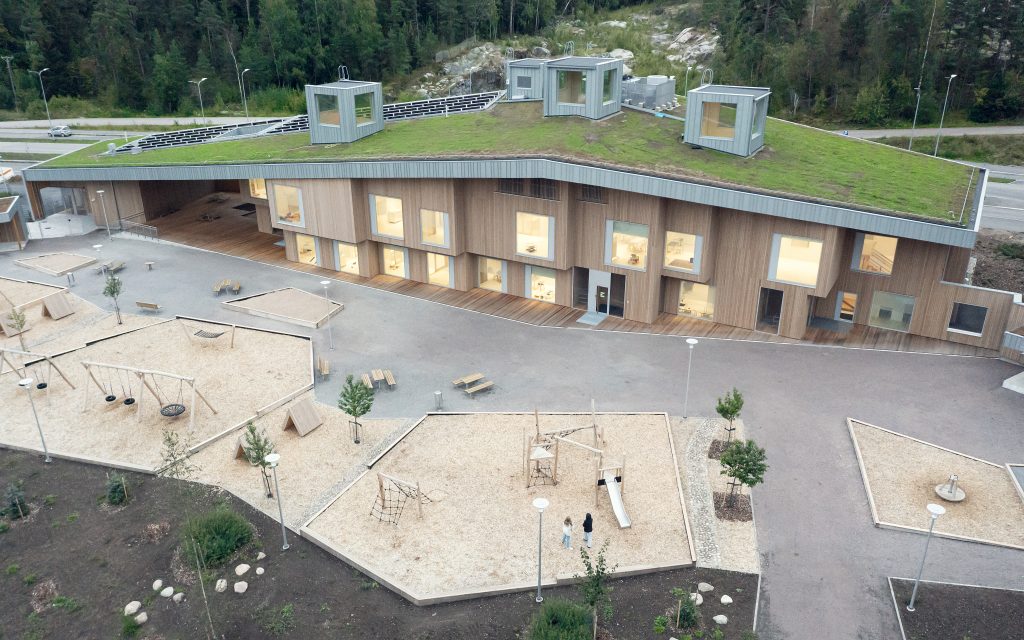
Different hybrid structures such as wood-concrete composites have gained traction in recent years. These structures aim to leverage the best of both worlds, so to speak. For example, a building’s non-load-bearing external walls can be all wood with a load-bearing frame of either concrete or wood, as needed. The Finnish Ministry of the Environment’s procurement criteria recommendations for low-carbon construction also support combining building materials. These criteria provide guidance on using renewable and recycled building materials in public construction projects [10].
Structural types of sound insulating wooden structures
When designing educational facilities, structural designers typically benefit from the expertise of acoustic designers in the selection of structural types [3]. Each structural type’s dimensions are often not determined by technical strength requirements but by the sound insulation or fire resistance of the structure or, in the case of horizontal structures, the vibration and permissible lateral displacement.
In terms of air sound insulation, wood floor panels are about 15dB weaker than concrete floors of corresponding thickness. The cut-off frequency of the structure coincidence also influences the amount of sound insulation achievable. All of this depends on the mass and stiffness of the structure. The cut-off frequency in wood panel structures usually falls somewhere in the middle of the frequency range that is relevant for building acoustics (Figure 3). This degrades the sound insulation [8].
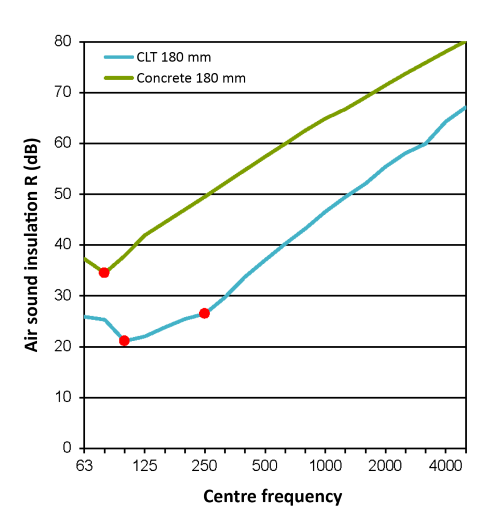
Figure 3. Air sound insulation by frequency in 180 mm thick CLT and concrete slabs, as calculated by a parametric air sound insulation calculation model. The red dot indicates the coincidence cut-off frequency; note that the CLT panel has two.
Wood’s lightweight nature and the resulting coincidence phenomenon mean that wood structures often need cladding to improve sound insulation. When lightweight partitioning walls are used as building load-bearing or stiffening structures, the wood frames and panels typically need a denser distribution of fasteners than usual. This combination can reduce the air sound insulation of the partitioning wall compared to a conventional steel frame of equivalent thickness. The sound insulation effects of the structural layers, air gaps, rigidity and many other factors of building components can be studied using parametric calculation models (Figure 3), which are accurate enough for design purposes [11–12].
Wood structure joints
In a wooden building, the chosen frame system has a significant impact on the structural lateral displacement and the sound insulation between spaces. There is currently no standardised or other generally accepted method for calculating losses at the joints of wood structures. In concrete structures, the sound insulation of joints is based on the stiffness of the joints and the mass of the associated building components. For wood buildings, these joints have largely been designed based on measurements and R&D for residential construction. [8-9]
Joint solutions developed for residential buildings are somewhat oversized for educational facilities because sound insulation requirements for classrooms are generally less demanding. Computational methods can be used to estimate the effect of structural lateral displacement on sound insulation [13]. However, no standard has evolved for joint solutions for wood construction. Rather, the structural breaks and sound insulation cladding needed to limit structural lateral displacement are calculated on a case-by-case basis.
Based on recent research, it would appear that structural breaks might not be necessary in educational facilities when the sound pressure level difference DnT,w between rooms is around 40-48 dB [14]. Most school spaces such as classrooms, office rooms, and student care facilities fall within this range.
Room acoustics in wooden educational facilities
Appropriate acoustic conditions in rooms enable effortless communication between teachers and students. When acoustic designers design educational facilities, their tasks include specifying room acoustic requirements and room acoustic structures and materials in collaboration with the architects. One example is the lobby of the Finnish-Russian school where room acoustics cladding has been stashed behind the widely-spaced wood latticework on the walls [3]. The aim was to leave as many load-bearing wooden structures as possible visible in the lobby (Figure 4).
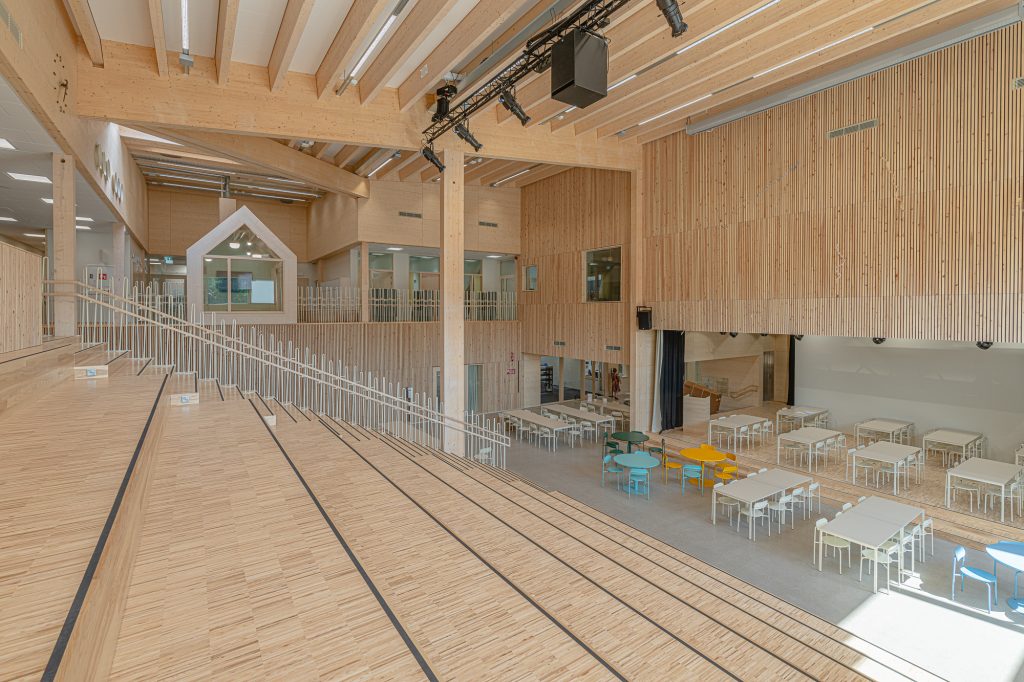
Wood buildings typically aim for big expanses of wood surfaces as an important part of their interior design. There is also a preference for wood-based materials in other interior cladding surfaces such as suspended ceilings and walls. Practical solutions include various wood fibre panels and perforated wood panels and latticework installed over a sound-absorbing material. Surface class requirements for surface materials in public spaces impose the most significant limitations on using wooden surfaces. The surface class of non-fireproof wood structures is generally D-s2-d2, excluding wood latticework structures, which have a lower surface class [15].
The interior cladding material in wooden educational facilities needs to be absorbent because, contrary to popular belief, wood either almost perfectly reflects sound like concrete or glass (solid wood layers) or absorbs sound mainly at low frequencies in the bass range (panel structures with air space behind them). The ability of building materials to absorb sound, in other words to reduce the volume of sound reflected from a material surface, is measured by the absorption ratio α. A value of 0 means that the sound completely bounces off the material without being attenuated at all. A value of 1 means that the material absorbs the sound completely and nothing bounces off.
Figure 5 shows the absorption ratio of a variety of surfaces. To get the absorption ratio needed to ensure speech is audible and clear or to significantly reduce the overall noise level in lobbies and canteens, wood surfaces need to be supplemented by either perforated panels or porous layers of material that improve the room’s acoustic conditions.
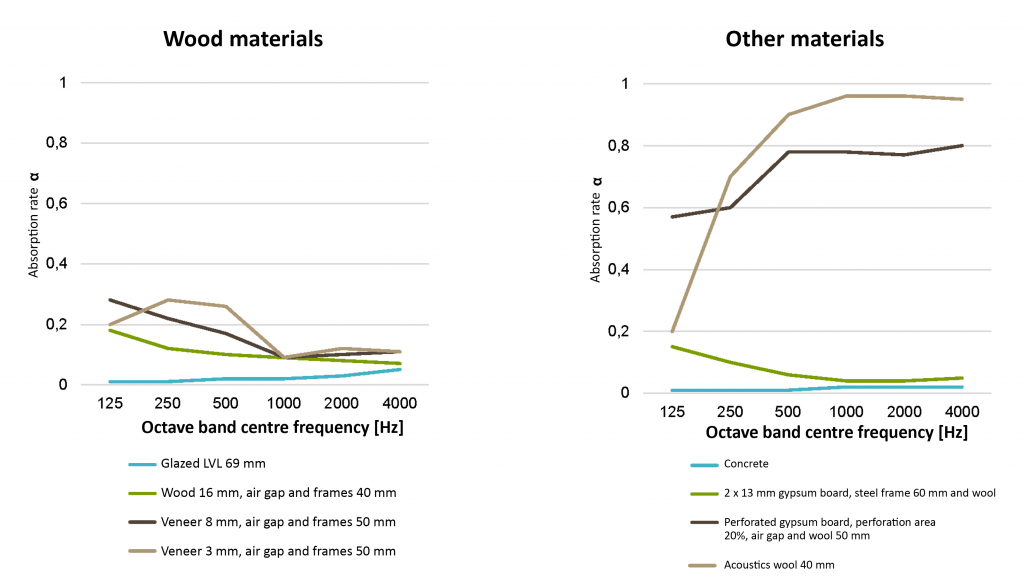
The opportunities presented by digital acoustics laboratories
Frame systems and structural types for wooden schools are not yet standardised. On the bright side, this means that there is room for development and optimisation. Research and development on sound insulation for wood structures began in Finland in the mid-1990s when building regulations finally allowed the construction of wooden buildings more than two storeys high. R&D was completely experimental at the time. Researchers used research literature or databases compiled from measurement results to figure out how different structural changes might affect the impact or air sound insulation between individual building components or spaces. They then further tested the acoustic performance of the structures either in the laboratory or in an experimental building [16].
In wood building construction projects, designs have largely been iterations of previously proven solutions. However, design or R&D that revolves around laboratory testing or experimental buildings can hinder the progress of wood construction. To help understand the problem, let’s take look at what happens in structural design: if structural design were based only on load testing of load-bearing structures with no calculations available for checking structural behaviour, the inefficiency would cost time and money.
Sound insulation measurements are time-consuming, and it is practically never possible to run extensive enough laboratory tests to optimise solutions. It has also been found that structures may not work the same way in real-life as they do in laboratories: connections to surrounding structures etc. affect a building’s sound insulation, and this cannot be replicated in laboratories. Constructing an experimental building is the other traditional option, but this is very expensive. It would make sense to use experimental building components or volumetric elements in actual buildings, but that is not always possible.
Calculation methods with different accuracy and speed levels have been developed in recent years to determine air and impact sound insulation. Parametric calculation models [11, 12, 17] are particularly useful as design tools in everyday construction projects. Numerical methods can be used for more demanding design tasks and for product R&D on structures because their accuracy best matches the uncertainty around sound insulation measurements. While there have been many proposed analytical calculation models for determining air and impact sound insulation, they have many boundary conditions that limit their usefulness. Even small changes in the structures being calculated may make the method inapplicable if the boundary conditions are no longer met. Numerical methods are not limited in this way [17].
Numerical methods can be used to check sound insulation by employing Finite Element Methods (FEMs), which are not limited by the boundary conditions typical for parametric or analytical methods. Traditionally, the use of Finite Element Methods in building acoustics has been limited mainly to low-frequency studies. This is probably due to the amount of computational power required for higher frequency acoustic models. Fortunately, modern day computers are up to the task. The opportunities provided by FEM modelling have been studied in wood construction in particular over the last 10 years. FEM methods can take the exact geometry of the structure into account and also include more complex material models, contacts and supports, which have more varying effects and implementation methods in wooden structures than in concrete [17–18].
FEM methods can accurately model the geometry of structures, joints and seals, which means they can also be used to model the air sound insulation of doors, windows, or log walls. This is something analytical or parametric models cannot do (Figure 6).
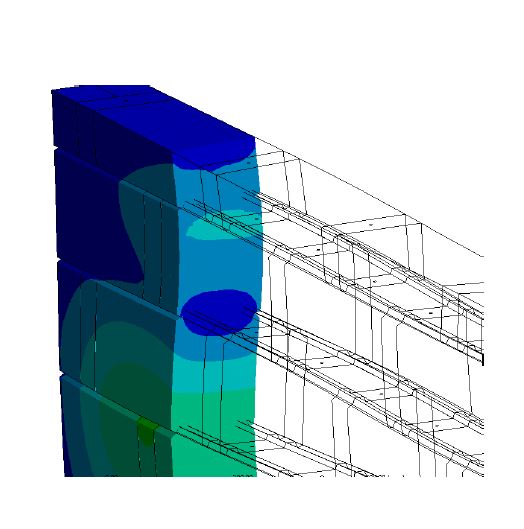
FEM methods have also proved useful in modelling the air and impact sound insulation of a wooden ribbed slab (Fig. 7). [17–18]
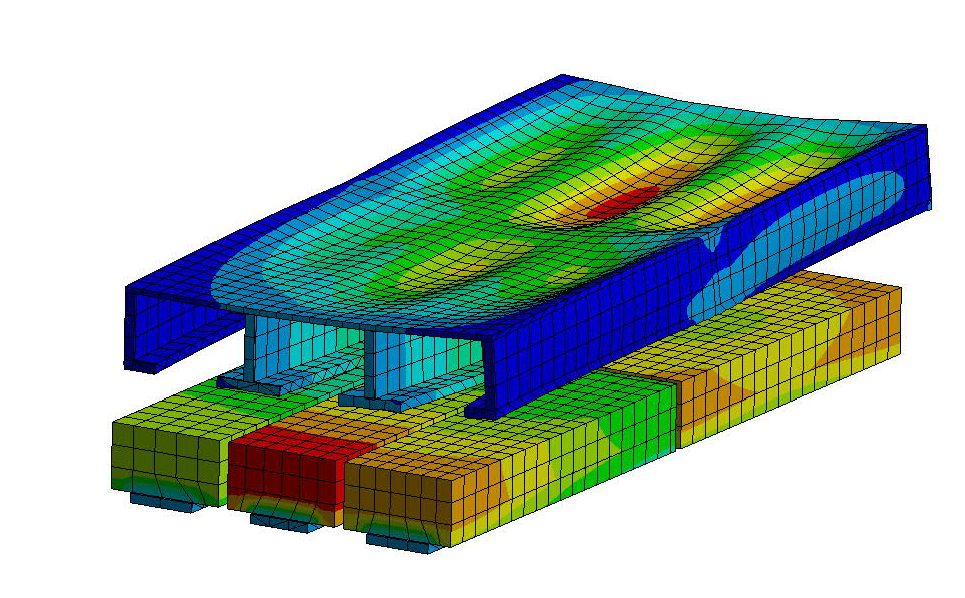
Edellä kuvatuilla mallinnusmenetelmillä voidaan rakenteiden ja materiaalien käyttäytymistä tutkia samalla tavalla kuin The modelling methods described above enable the study of structural and material behaviour just like in a laboratory, but faster and cheaper. In addition to air and impact sound insulation, FEM modelling can be used to precisely determine the sound attenuation characteristics of the dampeners used for attenuating noise from fans or airflow in ventilation ducts or industrial plants. The model can also be used to calculate absorption ratios for room acoustic claddings. Because these calculations are done digitally through modelling, the entire approach has become known as a digital acoustics laboratory. The construction products industry has seized on its potential and applied it broadly in design projects and product development projects alike.
CREDITS
The authors of the article, Mikko Kylliäinen, Pekka Latvanne and Antti Mikkilä, work in the acoustic design unit of A-Insinöörit. Kylliäinen is head of the acoustic design unit, a doctor of engineering, and also works as a postdoctoral researcher at Tampere University. He has worked on wooden building acoustics since 1995. Latvanne is a project manager and specialises in acoustic design for public wooden buildings. Mikkilä is a design manager who has served as chief acoustics designer in a number of public construction projects, including schools, hospitals, and libraries.
Mikko Kylliäinen Pekka Latvanne Antti Mikkilä
References
- Ympäristöministeriön asetus 796/2017 rakennuksen ääniympäristöstä.
- Kylliäinen, M. & Pääkkönen, R. 2017. Ääniolosuhteet avoimissa oppimisympäristöissä. Akustiikkapäivät 2017. Espoo, 24.–25.8., Akustinen Seura ry, pp. 21–26.
- Latvanne, P. & Mikkilä, A. 2021. Puurakenteisten oppilaitosten akustiikkasuunnittelun erityispiirteitä. Akustiikkapäivät 2021. Turku, 24.–25.11., Akustinen Seura ry, pp. 182-183.
- Kylliäinen, M., Björkman, J. & Hakkarainen, J. 2015. Akustisesti toimivan kustannustehokkaan puuvälipohjan kehittäminen. Rakennusfysiikka 2015. Tampere, October 20-22, Tampere University of Technology and the Finnish Association of Civil Engineers RIL, pp. 573-578.
- Latvanne, P. & Kylliäinen, M. 2017. Puuvälipohjien askelääneneristävyyteen vaikuttavat tekijät. Akustiikkapäivät 2017. Espoo, 24.–25.8., Akustinen Seura ry, pp. 117-122.
- Lietzén, J., Miettinen, J., Kylliäinen, M. & Pajunen, S. 2021. Impact force excitation generated by an ISO tapping machine on wooden floors. Applied Acoustics. Vol. 175, article 107821
- Lietzén, J., Kylliäinen, M. & Pajunen, S. 2021. Lattianpäällysteiden toiminta puuvälipohjilla. Akustiikkapäivät 2021. Turku, 24.–25.11., Akustinen Seura ry, pp. 66-71.
- Lahtela, T., Kylliäinen, M., Lietzén, J., Kovalainen, V. & Talus, L. 2021. Ääneneristys puutalossa. Helsinki, Puuinfo Oy.
- Kylliäinen, M., Latvanne, P., Kuusinen, A., Kekki, T. 2017. Puukerrostalojen ääneneristys: Expert report. Joensuu. Karelia University of Applied Sciences, Karelia-ammattikorkeakoulun julkaisu C44.
- Kuittinen, M. & le Roux, S. 2017. Vähähiilisen rakentamisen hankintakriteerit. Helsinki, Finnish Ministry of the Environment.
- Riitakangas, J. 2020. Ilmaääneneristävyyden parametrisen laskentamallin validointi. Bachelor’s thesis. Kuopio, Savonia University of Applied Sciences, degree program in construction technology.
- Latvanne, P. & Kylliäinen, M. 2019. Puuvälipohjien askelääneneristävyyden laskennallinen arviointi. Puu. Issue 3, pp. 42–50.
- Pura, M., Kylliäinen, M. & Kovalainen, V. 2021. Rakenteellisten sivutiesiirtymien laskennallinen tarkastelu puurakennuksissa. Akustiikkapäivät 2021. Turku, 24.–25.11., Akustinen Seura ry, pp. 84-89.
- Pura, M. 2021. Äänen rakenteelliset sivutiesiirtymät puurakennuksissa. Master’s thesis University of Oulu, Construction and urban engineering degree program.
- Lahtela, T. 2021. Paloturvallinen puutalo. Helsinki, Puuinfo Oy.
- Keronen, A. & Kylliäinen, M. 1997. Sound insulating structures of beam-to-column framed wooden apartment buildings. Tampere, Tampere University of Technology, Laboratory of Structural Engineering, Publication 77.
- Kylliäinen, M., Lietzén, J. & Kovalainen, V. 2021. Puurakenteiden ääneneristävyyden nykyaikaiset laskentamenetelmät. Rakennusfysiikka 2021. Tampere, 26.–28.10., Tampere University and Kiinko Kiinteistöalan Koulutuskeskus Oy, pp. 471–476.
- Lietzén, J. & Kovalainen, V. 2021. Rakenteiden ilmaääneneristävyyden arviointi elementtimenetelmällä. Akustiikkapäivät 2021. Turku, 24.–25.11., Akustinen Seura ry, pp. 102-107.